Home
TISSUE MIMICKING
Trying to mimic chameleon-like skin with a synthetic material presents scientists with a twofold challenge: The material must change color and must possess skin’s ability to go from a soft, compliant state and rapidly stiffens to prevent tearing. Here we design a triblock copolymer with linear ends and a bottlebrush middle block. The polymer forms physically cross-linked network that is soft, stiffs upon deformation, and scatters lights of different hues.
SHAPESHIFTING MATERIALS
Encoding the temporal evolution and reversibility of shape transformation is vital for minimally invasive surgery, drug release, and soft robotics. However, shapeshifting materials to date are irreversible and/or require external triggers, such as temperature, pH, light, chemicals, and sound. This conventional prerequisite limits viability in closed or inert systems and thus presents the need to develop materials that can change shape autonomously and in accordance with pre-determined rates and sequences.
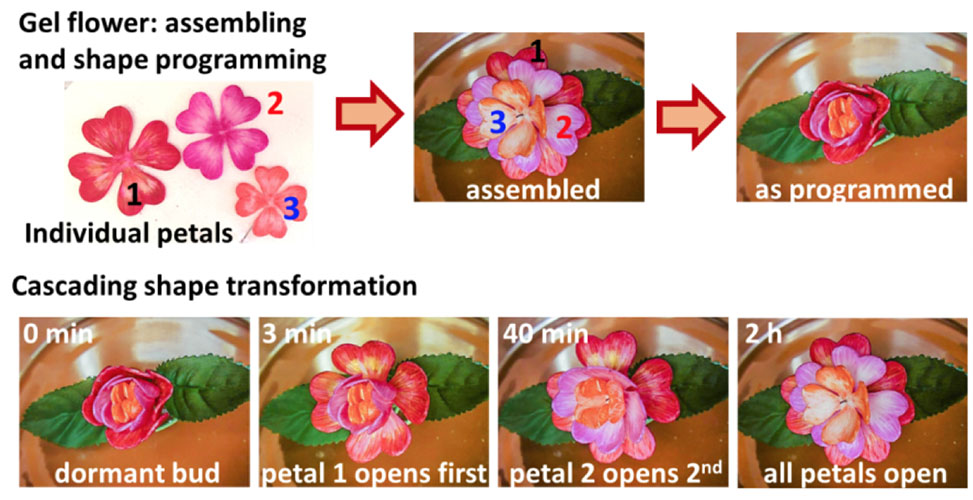
POLYMER ACTUATORS
In nature, muscles allow us to lift heavy objects, travel great distances, and accomplish detailed work such as sculpting and painting. To design artificial muscles that surpass, or at least mimic, those found in nature, we must first develop materials that efficiently convert electrical energy into mechanical work while also being intrinsically soft, flexible, and strong.
BOTTLEBRUSH MELTS AND ELASTOMERS
Advances in medical implants, tissue engineering substrates, synthetic membranes, and soft robots require materials that are soft, flexible, and tough. To satisfy this unmet technological requirement, our lab has developed a novel materials design platform. Specifically, we utilize precise control of branched polymer architectures to form polymer networks with encoded physical properties and property correlations.
POLYMER GELS
Unlike many living tissues, conventional synthetic hydrogels are inherently soft and brittle. In recent years, several strategies have been developed through incorporation of strong sacrificial bonds that allow for significant increase of both strength and deformability of hydrogels, including double-network, nanocomposite, and polyampholyte hydrogels. However, it remains challenging to combine high rigidity, high strength at break, high extensibility, and high elasticity.
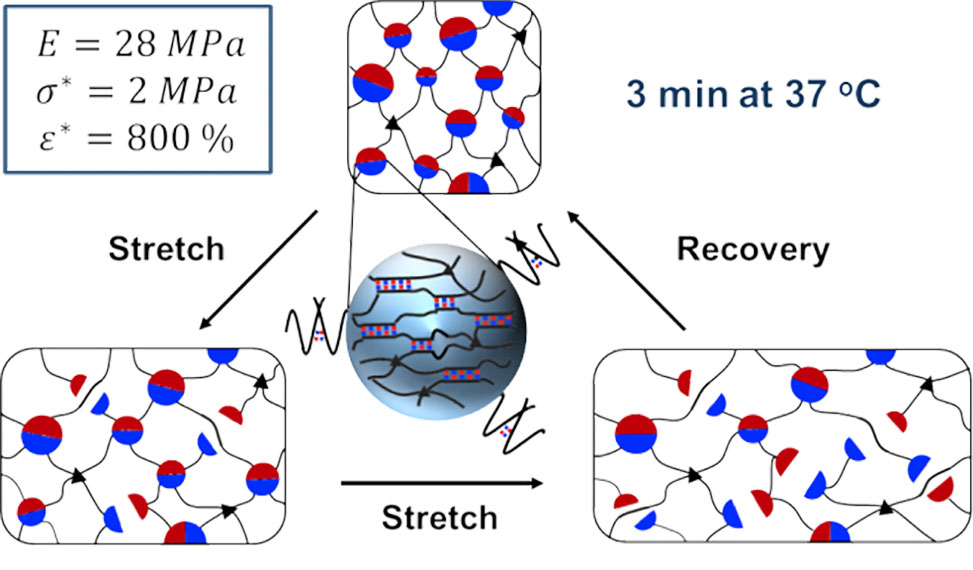
ENCAPSULATING AGGRESSIVE REACTANTS
Micro and nanoscale polymeric capsules are vital for an impressive array of applications. Whether as transporters, storage containers, or responsive vehicles, these structures impact drug delivery, energy storage, and sensor applications. Further, they are vital for the cosmetic, food, paint, construction, and oil industries. Different applications require different capsules, characterized by distinct geometrical, mechanical, and compositional combinations, and thus inspire work by our lab to provide solutions to the outstanding problems in the field.
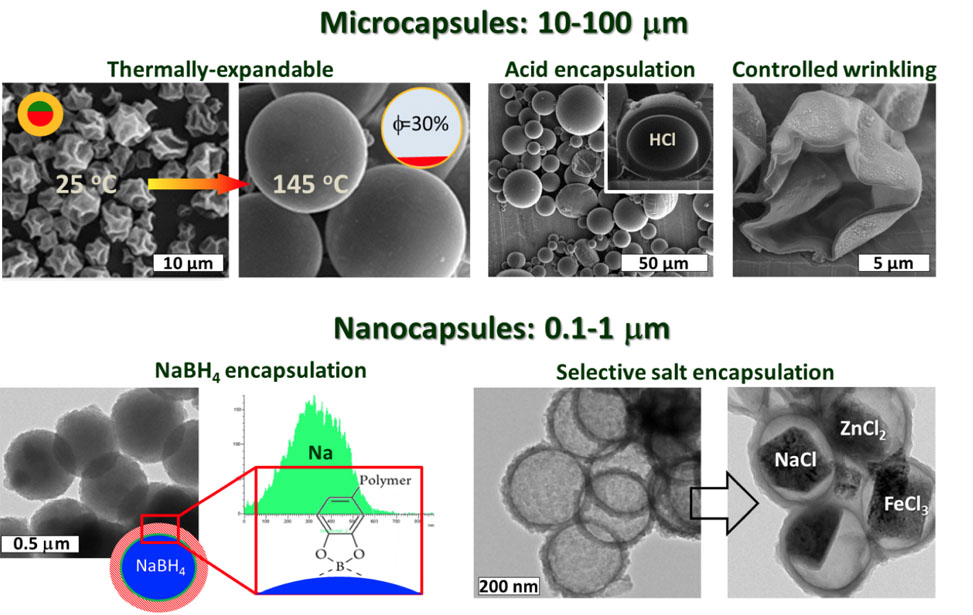
Sergei S. Sheiko
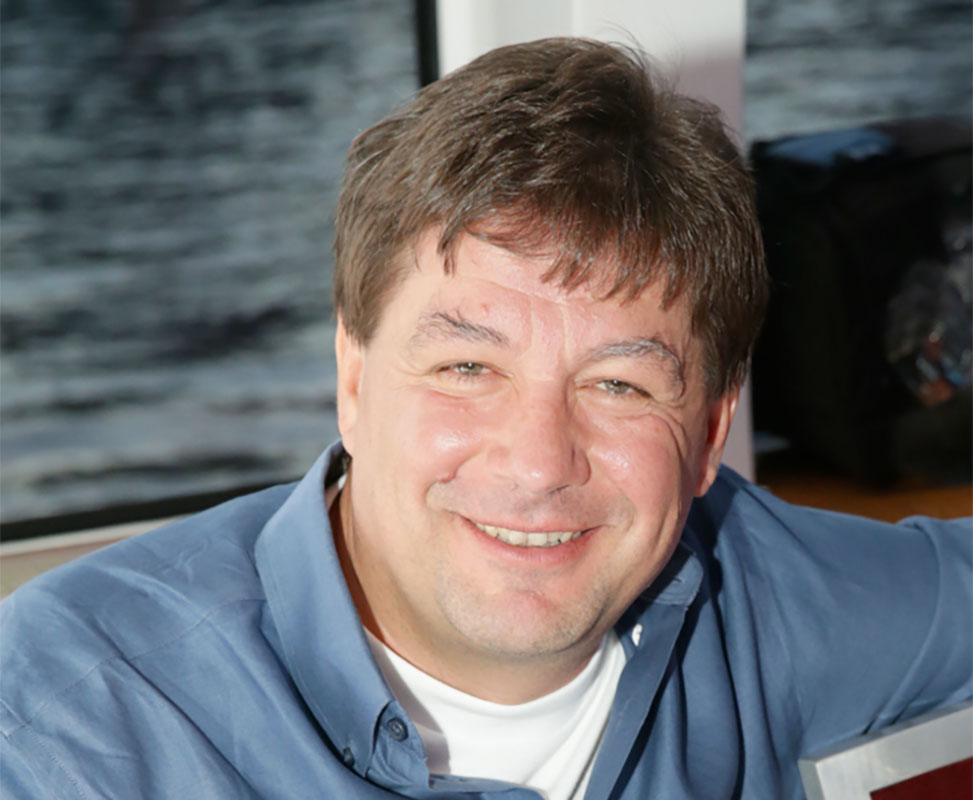
Professor Sergei Sheiko, Ph.D., Habil, aims at the design of novel polymeric materials with unique properties for biomedical implants, soft robotics, and enhanced oil recovery. He is currently focused on time-programmable materials with architecturally encoded physical properties.
Sergei is a George A. Bush, Jr. Distinguished Professor of Chemistry and Fellow of the American Physical Society.
Before joining the Chemistry Department at the University of North Carolina in 2001, Sergei has been working on his Habilitation in Polymer Chemistry at the University of Ulm in Germany and as Postdoctoral Fellow at the University of Twente in The Netherlands. He received a PhD degree in Polymer Physics from the Russian Academy of Sciences in 1991 and BS in Molecular and Chemical Physics from the Moscow Institute of Physics and Technology in 1986.
NSF Grant for MIRT
UNC Chapel Hill has received a $3.18 million National Science Foundation grant to establish a Materials Interdisciplinary Research Team, MIRT!
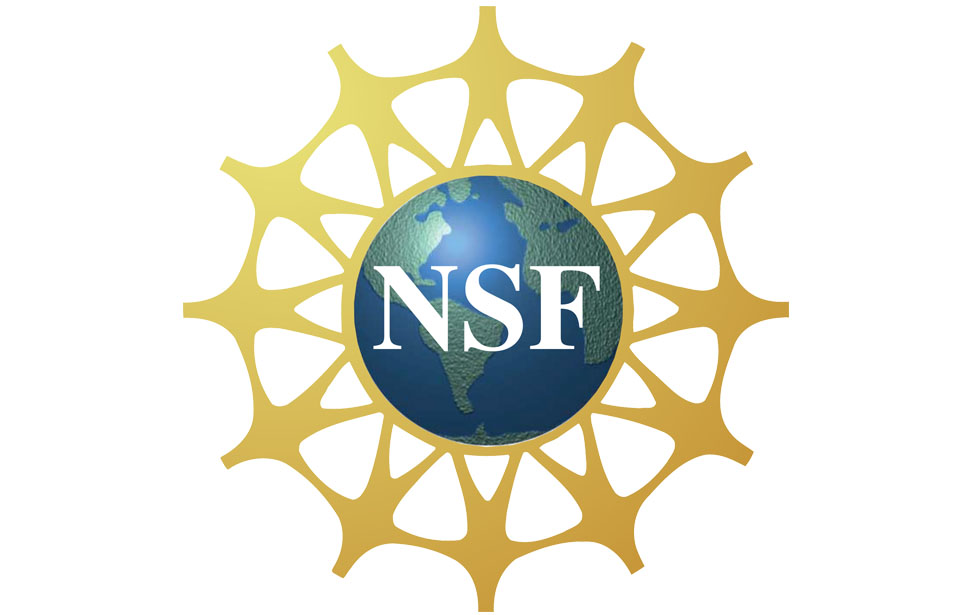
Some areas of research funded by this grant include thin polymer films, surface properties, wetting phenomena, molecular assembly, and the behavior of single molecules. Currently, the MIRT team is focused on the design of molecular tensile machines, exploring new strategies in lithography, developing molecular fluidics, and mechanical activation of specific chemical bonds at interfaces.
Using molecular imaging techniques we are able to observe how individual molecules move, self-organize, respond, and react on surfaces. These studies have direct implications on microelectronics, photonics, fluidics, and oil recovery, for example, technologies that greatly exploit the microstructure and surface properties of thin polymer films.